Ultracold Ytterbium in dynamic potentials
The Ytterbium Experiment
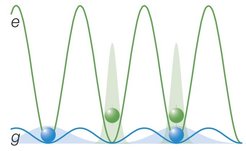
Our experiment traps and cools Ytterbium (Yb) atoms. Ytterbium is a rare earth element with an electronic configuration corresponding to that of Alkaline-Earth elements. Compared to Alkali elements, which are the most common choice in ultracold gas experiments, these atoms have one additional electron - making them a bit more complex, and a lot more versatile.
One of the most striking properties of such elements is the fact that there are two electron spin configurations, singlet and triplet. Just as in Helium, the ground state has the singlet configuration, and transitions between singlet and triplet states are suppressed. This means that the lowest energy state with a triplet configuration is also stable, because it can not decay! Remarkably, however, it has a much higher energy than the ground state, with an optical transition wavelength of 579nm in the case of Yb. Due to the very weak coupling on the transition, this wavelength has been used for example for optical frequency standards in the most precise clocks.
We are interested in this singlet-triplet splitting due to the fact that the atoms have very different properties in these two states - both in the way how they interact with light and the way they interact with each other. By changing the internal state, we can therefore change the behavior of the atoms, and there can even be both states present at the same time. This allows for the generation of very interesting many-body Hamiltonians, such as for example those related to Kondo physics in condensed matter physics.
A second property of cold alkaline earth atoms is the fact that in their ground state, the different nuclear spin sublevels are extremely decoupled from the electron shell, and therefore are basically indistinguishable - the resulting description in a Hamiltonian is hence symmetric with respect to the spin, just as in the usual case of a spin 1/2 system - except that this is now generalized to a higher spin, for Yb up to 5/2, with 6 separate sublevels.
Yb setup
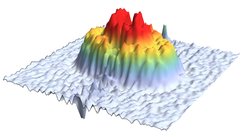
In our setup, we laser-cool hot Ytterbium, which is first evaporated in an oven at 400°C, to about 20 microkelvin absolute temperature, and further cool it to quantum degeneracy by evaporative cooling.
We mostly work with the isotopes 173Yb (a fermion) and 174Yb (a boson).
Laser cooling is achieved with two separate lasers: First, atoms from the oven are slowed using exclusively the strong 1S0-1P1 transition. For obtaining low temperatures, we cool with a MOT on a narrow intercombination line in the green range of the spectrum (555.6nm). As the bosonic isotopes have no Zeeman sublevels, there is no simple sub-Doppler-cooling effect.
Fermions
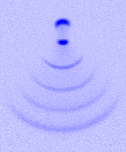
Our main interest is with fermionic Ytterbium, which we can cool to degeneracy and load into lattices. The Fermion has six substates, which we measure separately with a Stern-Gerlach technique. In order to prepare the atoms in the two separate "orbitals", the singlet and the triplet state, we have a clock laser, which allows for coherent coupling of the ground state and the 3S0 triplet state on the extremely weak clock transition. We are pursuing several interesting aspects of many-body physics with alkaline earth-like atoms, and at the same time, discover new ones. While we were exploring the properties of the interactions between the ground state and the metastable state of the system, for example, surprising scattering properties were found, which led to the prediction and later discovery of a Feshbach resonance with a novel structure. This resonance gives rise to interesting physics for strongly interacting particles, and allows for tuning the strength of the interactions between certain states of the atom. By using the atoms as an extended analogue to electrons in a crystal lattice, ytterbium also allows us to do many-body physics in novel ways: The extended SU(N)-symmetry of the atoms gives rise to generalized models for metals and insulators, and even magnetism. Using modern methods of probing the atoms in the trap, we can directly observe the changes in the equation of state of the gas, literally "seeing" the transition from a metal to an insulator, for example.
Recent Selected Publications
- +49 89 3 29 05 - 238 (MPQ)
- +49 89 3 29 05 - 313 (MPQ)
- +49 89 2180 6126 (Office) // -6136 (Lab) //
- +49 89 2180 63851